Understanding Glyphosate: Persistence in Soil and Impact
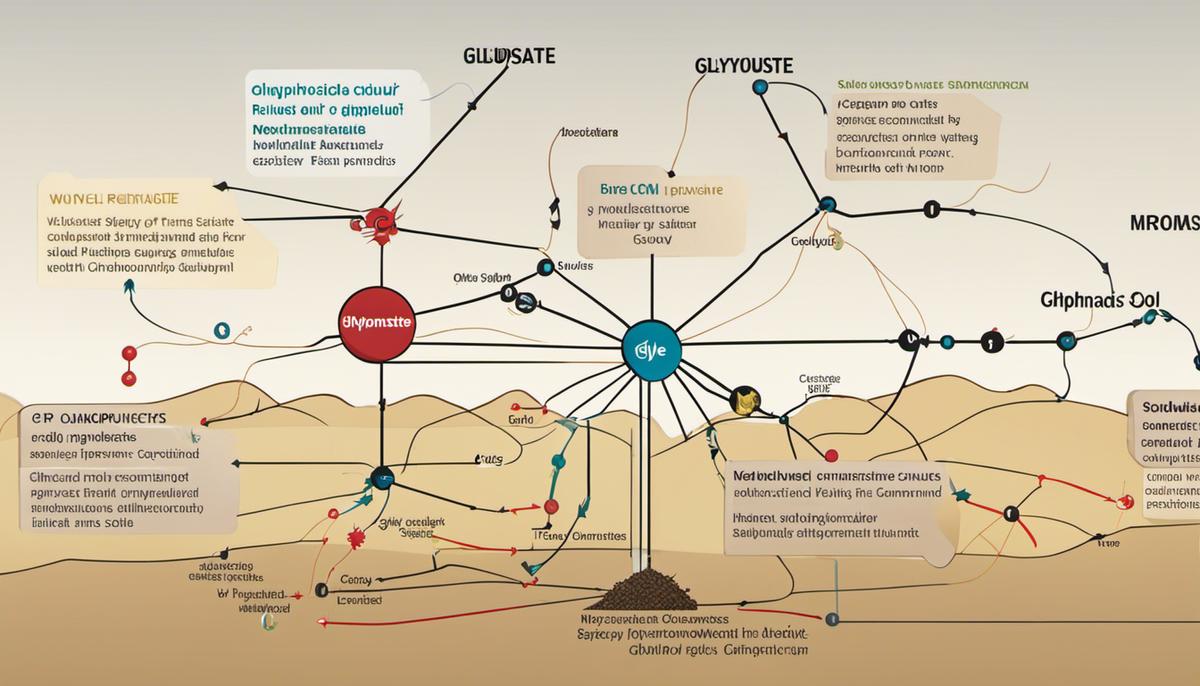
Understanding the environmental impacts of glyphosate is fundamental to its responsible and sustainable use. Glyphosate, a common yet controversial weed killer, commands global attention due to its omnipresence in agricultural practices and the potential health hazards it poses. This exploration delves into the chemical structure and function of glyphosate, focusing on why its characteristics lead to a persistent presence in soil environments. It highlights the various factors influential in glyphosate’s breakdown process, including local environmental conditions, soil characteristics, and microbial activity. Giving special consideration to the methods of detecting glyphosate residue, the exploration illustrates modern science’s contribution to understanding its persistence. Furthermore, the often overlooked environmental and health impacts of glyphosate are also discussed comprehensively. Wrapping up the discourse, mitigation strategies and safer alternatives to glyphosate are introduced, promoting sustainability and environmental protection.
Chemical Structure and Function of Glyphosate
Unraveling Glyphosate: A Fascinating Exploration of Its Chemical Structure’s Influence on Function and Soil Persistence
In the sphere of herbicides, few have gained as much notoriety and academic interest as glyphosate. Introduced in the 1970s and best known as the active ingredient in Roundup®, glyphosate has become the most frequently deployed herbicide worldwide. This prevalence, alongside increasingly heated debates surrounding potential side effects, has ignited a scientific quest to thoroughly understand the intricacies of this compound. This article aims to elucidate upon the fascinating aspects of glyphosate’s chemical structure and how it contributes to its crucial function and persistence in soil.
Glyphosate, chemically recognized as N-(phosphonomethyl) glycine, owes much of its herbicidal effectiveness and environmental resilience to its unique chemical structure. Bearing both a carboxyl group (-COOH) and a phosphonic acid group (-PO3H2) flanking a central carbon atom, glyphosate is a potent chelator, capable of forming strong bonds with a variety of cations, particularly divalent metal ions such as calcium, magnesium, and iron.
The chelating potential, an inherent attribute of glyphosate’s unique structure, underpins its primary function as a non-selective, broad-spectrum herbicide. Glyphosate finds its target by inhibiting the shikimate pathway in plants and some microorganisms, fundamentally interfering with the biosynthesis of essential amino acids phenylalanine, tyrosine, and tryptophan. Glyphosate’s molecular design allows it to sturdily bind to the enzyme EPSP synthase, critical for the shikimate pathway, thereby rendering this pathway inoperative and leading to the eventual death of the plant.
Glyphosate’s persistence in soil, however, is less a product of its molecular structure and more of a concert between its chemical properties and external soil factors. Importantly, glyphosate’s phosphonic acid group renders it a highly polar molecule. Polar molecules exhibit a vibrant tendency to bind to particulate matter in the soil, an interaction potentiated by glyphosate’s inherent chelating properties. The ensuing bond between glyphosate and soil matter is incredibly strong, resisting degradation and restricting glyphosate’s mobility within soil strata.
Nevertheless, the interaction between glyphosate and various soil components is highly dynamic and contingent on soil type, pH levels, and presence of metal ions. Under specific conditions, glyphosate may transition into a less polar state, enhancing its potential for leaching and maintaining its persistency within the environment.
Insights into glyphosate’s chemical structure elucidate its function and persistence in soils, underscoring the intricate harmony between a molecule’s design and its ensuing behavior within the environment. However, further studies are required to comprehend the myriad factors and subsequent interactions that influence glyphosate’s behavior in diverse soil ecosystems. As we continue to apply glyphosate at scale across agricultural landscapes, a comprehensive understanding of this molecule and its properties becomes ever more critical for our ongoing stewardship of the environment.
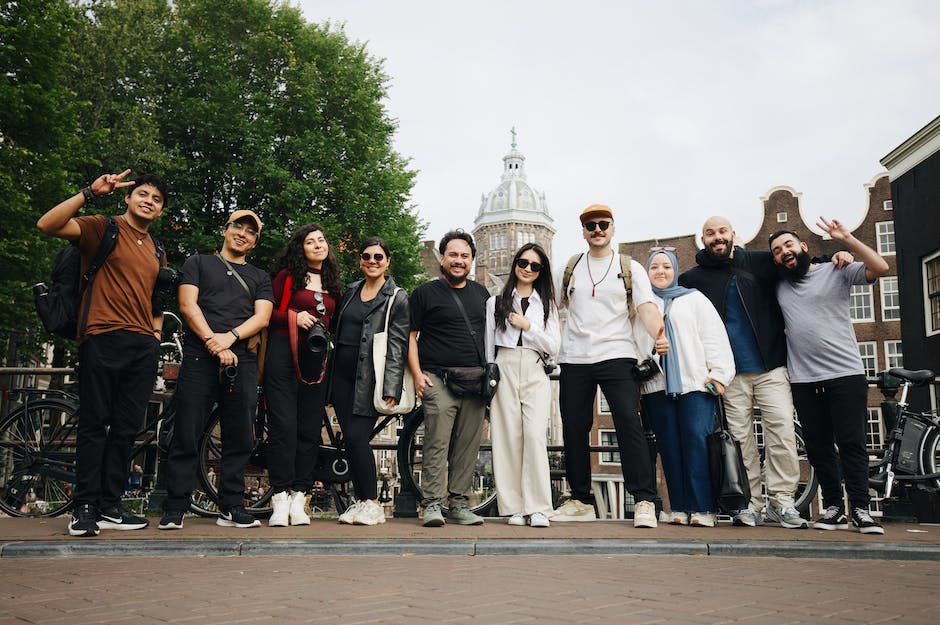
Factors Influencing Glyphosate Breakdown
The mechanisms of glyphosate degradation primarily entail microbial utilization and chemical modifications. These are influenced by three significant categories of external factors that can hasten or impede these processes: environmental conditions, physical soil properties, and chemical variations in the soil.
Environmental temperatures play a pivotal role in glyphosate degradation. With a rise in temperature, the metabolic activity of soil microbes responsible for glyphosate degradation generally increases, accelerating the process. Ambient temperatures for optimal degradation range between 30°C and 40°C. Outside these limits, the degradation speed declines. Precipitation also affects these rates, with higher moisture levels increasing microbial activity and thus catalyzing the process. However, excessive rainfall can lead to increased leaching, potentially allowing glyphosate to enter groundwater sources before degradation occurs.
Physical soil properties, such as texture and composition, significantly influence glyphosate’s degradation. Clay-based soils with a higher surface area and porosity bind the herbicide tightly, delaying microbial degradation. On the other hand, loamy and sandy soils with lower clay content may facilitate faster degradation due to the less effective adsorption to soil particles, although these types of soil can also lead to leaching.
Ultimately, the chemical nature of the soil also determines the rate of glyphosate degradation. High pH levels can increase the resistance of the glyphosate molecule to degradation. As glyphosate is a strong chelating agent, soils high in iron, aluminum, or manganese can slow the herbicide’s degradation as it binds to these elements. By contrast, soils with high phosphorus levels can enhance glyphosate’s dissolution, promoting quicker microbial utilization and degradation.
The presence of particular soil microorganisms is essential for glyphosate degradation as well. This process often begins with the cleavage of the carbon-phosphorus bond in the glyphosate molecule by microbial enzymes, primarily glyphosate oxidoreductase and C-P lyase. The existence of certain bacterial species in soils, such as Pseudomonas sp, Bacillus sp, and Achromobacter sp, have been proven to degrade glyphosate effectively. Thus, the soil’s microbiological profile can greatly influence the rate of degradation.
These interacting factors culminate in a wide range of possible degradation rates for glyphosate, from as few as 3 days in ideal conditions to potentially decades in adverse circumstances. Devising mitigation strategies requires a deep understanding of the conditions most favorable for glyphosate degradation and how they interact in a given soil infrastructure. This topic certainly warrants continued exploration and research.
Due to its widespread use, the behavior of glyphosate in various ecosystems continues to be a topic of significant environmental interest. The consideration of the various factors that affect the rate of glyphosate breakdown— environmental factors, physical soil attributes, and soil chemical composition— can assist in minimizing the potential environmental and health impacts of this globally employed herbicide.
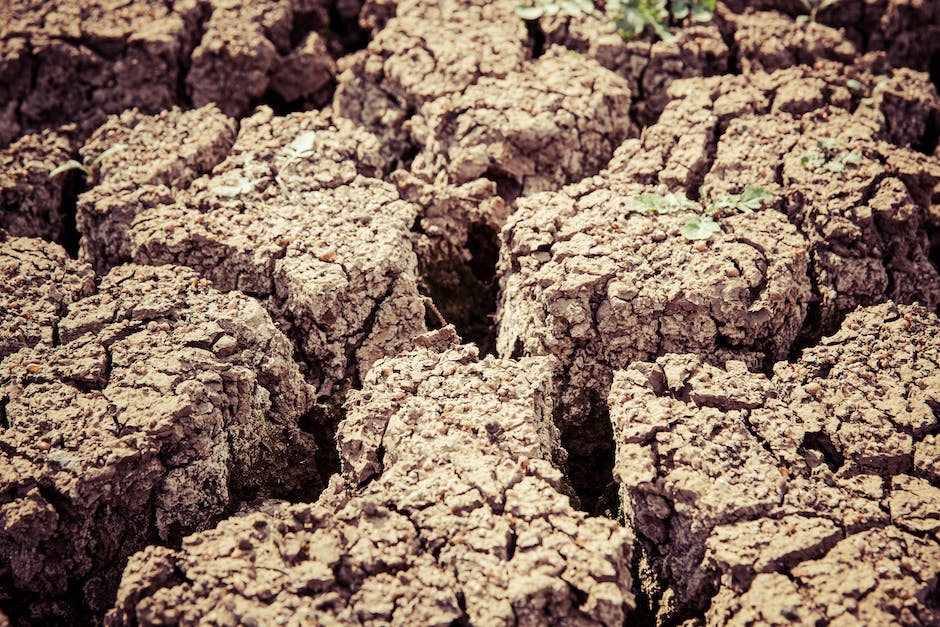
Testing and Detection of Glyphosate Residue
In advancing the discussion, it is practical to differentiate analytical methods utilized in detecting glyphosate residues in soil. Most notably, chromatographic techniques like High Performance Liquid Chromatography (HPLC) and Gas Chromatography (GC) predominantly serve as the cornerstone approaches, often coupled with mass spectrometry (MS) to enhance sensitivity and confirm detected residues.
High Performance Liquid Chromatography boasts numerous benefits, such as high sensitivity and capability for simultaneous detection of multiple contaminants, including glyphosate. Typically, the soil sample is first treated with an extraction solvent like Methanol or Acetonitrile, then surfactants are added to enhance glyphosate solubility. The extracted solution goes through a purifying process to remove potential interferences, and only then, chromatographic analysis commences.
Gas Chromatography, albeit less commonly used due to glyphosate’s high polarity and low volatility, has been proven effective in modified forms. Derivatization, for example, entails chemically modifying glyphosate to augment its responsiveness to GC, thus facilitating successful analysis. Evidently, GC-MS, with its excellent selectivity and sensitivity, harbors phenomenal amplifying effects for detecting glyphosate wherever present, albeit with the tradeoff of lengthier analytics.
As methodologies have evolved, robust techniques have surfaced centering on immunoassays (ELISA: Enzyme-Linked Immunosorbent Assay) and biosensors. Immunoassays are prominent in detecting low levels of glyphosate due to their sensitivity and specificity. This technique leverages specific immune reactions – between the glyphosate and anti-glyphosate antibodies – to indicate the presence of glyphosate. However, this method provides only qualitative information and might be less effective for complex soil matrices.
Biosensor technology, on the other hand, provides an innovative approach for rapidly detecting glyphosate. Based on an intimate understanding of enzyme kinetics, these sensors harness the reaction between glyphosate and a specific enzyme (for instance, glyphosate oxidoreductase), generating a readable signal in the presence of glyphosate. Researchers find this technology promising on account of its benefits like real-time monitoring and on-site analysis, albeit with a relatively lower sensitivity than chromatographic and immunoassay techniques.
Capillary Electrophoresis (CE) is another viable detection approach. This technique segments ions based on their electrophoretic mobility throughout a narrow capillary filled with an electrolyte. In detecting glyphosate, CE potentially renders high sensitivity and efficiency, in addition to allowing for simpler sample preparation and lower solvent consumption.
Considering the intertwining complexity of soils and their ecosystems, it becomes clear that the selection of an appropriate detection method is pivotal. Each has its own unique strengths and weaknesses, which could be influenced by external and internal soil factors. Hence, a comprehensive understanding of each technique merits itself to making informed decisions for an accurate evaluation of glyphosate presence.
The exploration of glyphosate and its impact on the biosphere is a persistently active field. Comprehensively examining glyphosate residues in soil allows for sound scientific conclusions on environmental health impact and the development of more sustainable agricultural practices. As research perpetually propels forward, methods can only be expected to enhance in their sensitivity, versatility, and efficacy.
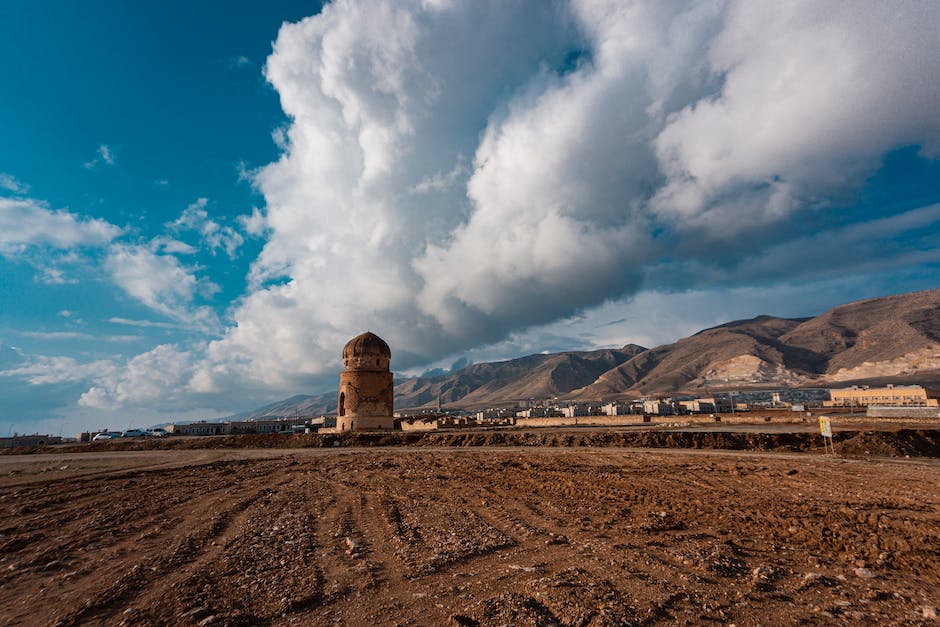
Environmental and Health Impacts of Glyphosate
Shifting from the intricate dynamics of glyphosate-soil interactions and detection techniques, we delve into the crux of why these investigations hold paramount importance: the environmental and health implications linked to persistent glyphosate in soil.
Glyphosate’s non-selectivity signifies that beyond targeted weeds, it potentially affects non-target organisms. Several environmental organisms and processes deeply intertwined with the biosphere’s overall health may inadvertently fall victim to glyphosate’s inhibitory pathways. The breadth of this collateral damage is yet to be fully conceived and quantified.
Terrestrial and aquatic ecosystems face potential risks due to a profound perturbation in biodiversity. Glyphosate, even in allowable limits, has shown to reduce the population and diversity of earthworms and other macrofauna instrumental in soil processes such as nutrient cycling and organic matter decomposition. Moreover, freshwater algae and invertebrates, critical for aquatic food webs, are suspected to suffer potentially fatal effects given high-enough exposure levels.
Glyphosate’s impact extends to the health of our pollinators. Multiple studies have documented its sub-lethal effects on honeybees, altering their behavior, learning, and memory, which in turn affects pollination dynamics. Glyphosate’s impact on microbes may disrupt the gut microbiota of bees, leading to increased vulnerability to pathogens and reduced overall health. The ripple effect of such changes in pollinator populations could dramatically alter agricultural production and ecosystem resilience.
Plant health and subsequent agroecosystems are far from immune. Glyphosate may interfere with symbiotic relationships, such as those between plants and mycorrhizal fungi – partnerships vital for nutrient uptake, resistance to pathogens and drought, and post-disturbance recovery.
From an anthropocentric lens, public health considerations are equally pressing. Glyphosate has the potential for bioaccumulation in edible plant tissue, leaching into ground and surface waters, widely used for drinking and irrigation purposes. WHO’s International Agency for Research on Cancer classifies glyphosate as “probably carcinogenic to humans,” with studies showing a potential association with non-Hodgkin lymphoma and childhood leukemia. However, it should be emphasized that scientific consensus around this concern is not yet solidified, reinforcing the need for continuous rigorous investigations.
Probable adverse health outcomes such as endocrine disruption, liver disease, and developmental effects have also been suggested, yet the lines of causality remain murky in human epidemiology due to multiple variables at play. Comprehending the direct and indirect pathways of exposure to glyphosate, coupled with factors influencing human susceptibility, requires interdisciplinary efforts to unravel this complex matrix.
The implication of glyphosate’s environmental persistence cannot be detached from the broader conversation around sustainable practices in agriculture and land management. Farmers, policy-makers, scientists, and consumers — all have pivotal roles to play. Informed decisions, promoting eco-friendly alternatives to glyphosate, stringent regulation, and public engagement in these concerns are the commitments our shared environment and public health demand of us.
Puzzling out the glyphosate enigma requires further study in light of its widespread global use. This necessitates research integrating numerous soil contexts, exposure pathways, non-target species, and human health interfaces. The enigma is not just glyphosate, but our readiness to confront the complexities of anthropogenic ecosystems with a stewardship that balances our needs with that of the multitude of organisms sharing our environment.
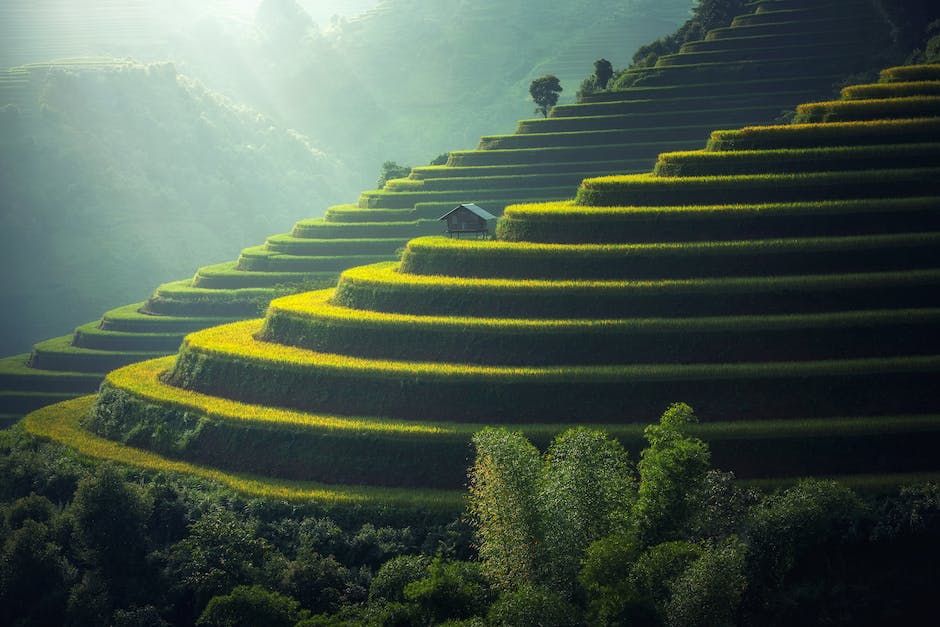
Mitigation and Safe Use Practices for Glyphosate
Glyphosate application, like any pesticide use, requires sophisticated knowledge and meticulous practice for maximum efficacy with minimal harm. Safe use practices for glyphosate entail equipping applicators with proper personal protective equipment to mitigate exposure risks. Understanding the principles of integrated pest management also holds the key to mastering judicious and sustainable applications of glyphosate, which includes monitoring pest populations, ascertaining the economic thresholds for intervention, and employing a variety of control methods.
The application timing significantly influences the glyphosate load in environmental matrices. Applying glyphosate during dry periods diminishes runoff potential, but coupling this with accurate forecasts can contribute to realizing this best-practice. Early morning applications, when dew softens the cuticle of the weeds, enhances absorption whilst circumventing evaporation losses due to the midday heat. Other operational parameters conducive to glyphosate’s efficacy pertain to the sprayer calibration, nozzle selection, water volume, and spray droplet size. Still, in order to maintain efficacy, thoughtful consideration of the aforementioned parameters is of utmost importance due to the risk of developing resistance.
Evidently, glyphosate is not a silver bullet solution; exploring other control strategies are essential. This calls for the adoption of non-chemical strategies, such as mechanical, cultural, and biological methods. Crop rotation and tillage, for example, interrupt weed life cycles and reduce weed seed banks. Cover cropping, or planting certain crops specifically to suppress weeds, are particularly encouraging as they possess a dual utility of drastic weed reduction and soil health improvement.
Promising pioneers in biological weed management include predatory insects, plant pathogens, and allelopathic plants, which have potential in sustainable weed suppression and disrupting the monopoly of a single-control method. Non-target organisms’ vulnerability to glyphosate highlight the pressing need for increased understanding and application of these strategies. In the face of burgeoning concerns, the potential of these alternative methods is tremendously high, granting further research and innovation efforts the opportunity to mitigate the risks born from heavy glyphosate usage.
Genetic engineering, despite contentious discourse, also offers an intriguing potential for favorable alternatives. The introduction of genes into cash crops that increase their competitiveness against weeds provides a feasible strategy. Genetically modifying crops to limit the amount of glyphosate they metabolize, and therefore pass into the environment, provides another imaginative solution.
In conclusion, the judicious use of glyphosate, the inclusion of alternative non-chemical tactics, and continued research and development of biology-based alternatives are amply required to mitigate the persistence and impact of this herbicide. Glyphosate, by virtue of its non-selectivity and broad spectrum of application, remains an indispensable tool in modern agriculture, albeit the escalating cautions to its populace culminate in a pivotal moment for an extensive paradigm shift in our understanding and management of agrochemical use.
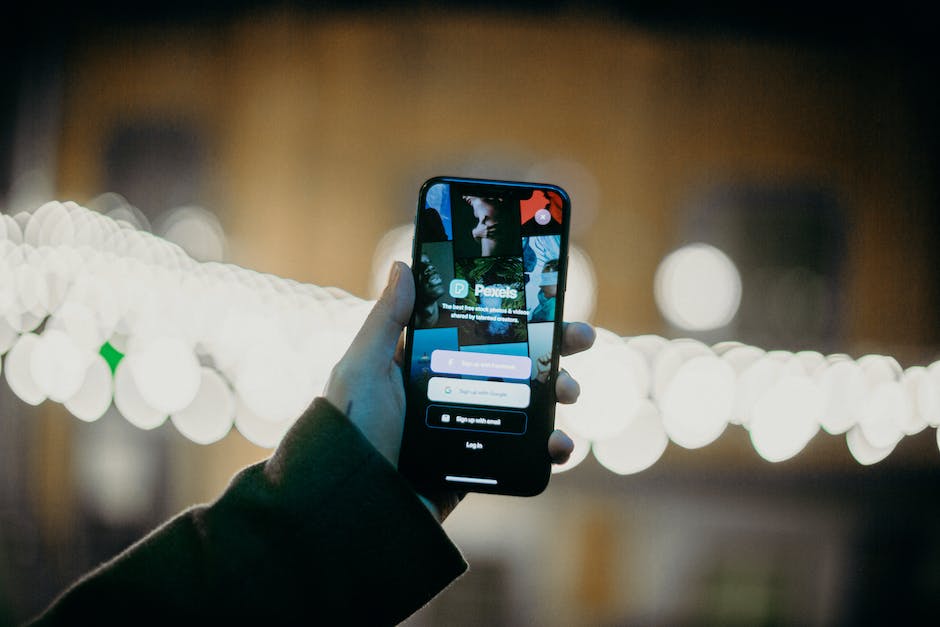
The intricate nature of glyphosate’s chemical structure lends itself to resilience, a trait that challenges both detection and mitigation strategies. Yet, without denial its potential risks to environmental and human health call for more careful, considerate, and informed usage. By employing advanced testing techniques and building awareness around intrinsic and extrinsic factors influencing glyphosate’s decay, the challenges it presents can be better managed. While it may seem like an uphill battle, the promotion and utilization of safer and more sustainable alternatives stand as a beacon of hope for pursuing a planet-friendly agricultural future. Therefore, the discourse must continue, centering on scientific exploration, raising awareness, guiding policy, and fostering innovation, thereby steering the world towards a more sustainable agricultural paradigm that balances productivity with the health of our fragile ecosystems.